Funded Projects (External and Internal)
- $199,786 National Science Foundation, CBET Division, PMP Program (#2347493), “ERI: Simultaneous Interactions between Gases, Liquids, and Adsorbents in Adsorbent-Coated Microchannels” Role: PI (Only PI)
- $2,050,000 Department of Energy Biological and Environmental Research, October, 2023, Award number: DE-SC0024701, "Biological routes for synthesizing the industrial platform chemical, propylene, from deconstructed lignin waste and captured carbon dioxide produced during lignin valorization into bio-oil", Role: Co-PI, (Share: $360,066)
- $11981 Provost Seed Grant on "Passive Roadway Deicing using Embedded Adsorbents" (Role: PI)
- $30,000 Institutional Research Incentive Award from Florida Tech, October 2022 – Cost-Effective and Atomic-Level Design of Metal-Organic Framework (M.O.F.) Adsorbents for Energy-Efficient Water-Based Thermal Systems (Role: Co-PI)
- $21,200 Institutional Research Incentive Award from Florida Tech, October 2022 –Improving Static Cold Storage Life of the Human Heart before Transplant (Role: Co-PI)
- $26,500, Institutional Research Incentive Award from Florida Tech, October 2021 – Carbon Dioxide Capture from the Flue Gas using a Novel Bio-derived Adsorbent (Role: PI)
- $34,130, Institutional Research Incentive Award from Florida Tech, October 2021 - Quantification of Anions and Cations using Ion Chromatography (Role: Co-PI)
High Performance Microchannel Adsorption Heat Pumps
The HVAC sector is dominated primarily by the vapor compression cycle. However, there are two primary concerns: first, they are driven by electricity and secondly, the refrigerants used in the vapor compression cycles have the potential to cause harm to the environment. Hence, widespread efforts are being directed toward the investigation of refrigerants with low ozone depletion potential as well as global warming potential, and also with low flammability and toxicity. Adsorption heat pumps have the potential to utilize waste heat and provide cooling and heating with minimal electricity use and few moving parts. Conventional embodiments of adsorption heat pumps restrict their applicability due to some key design limitations. The adsorption and desorption cycle times for such systems can be up to a few hours depending on the system size and working pair, making them impractical for applications such as space conditioning. Rapid cycling of the adsorbent beds increases the adsorbent system viability for HVAC applications by yielding a competitive performance. This research thrust involves the development of adsorption heat pumps incorporating adsorbent-coated microchannels, which allows a highly asymmetric operation and in turn near-continuous cooling.
Depending on requirements, adsorption and desorption processes in this adsorbent bed can be triggered. Furthermore, such a heat pump design can be easily implemented in automobiles, where the radiator coolant can become the source for actuation of the heat pump, thereby eliminating the need of a dedicated compressor.
Pahinkar, D. G., D. B. Boman and S. Garimella (2020). "High Performance Microchannel Adsorption Heat Pumps." International Journal of Refrigeration DOI: https://doi.org/10.1016/j.ijrefrig.2020.07.020.
Novel Adsorbent Coating Methods
Existing adsorption systems suffer from performance and scalability limitations; thus, significant improvements to the state-of-the-art are required. There are two major bottlenecks in terms of mass and heat transfer. The first is the widespread use of packed adsorbent beds, which exhibit low mass transfer rates. The second issue is the low thermal conductivity of the adsorbent material, making rapid heating and cooling of the adsorbent difficult. These two issues can be solved by using adsorbent-coated microchannels, which provide excellent heat and mass transfer characteristics. However, the methods to fabricate these microchannels are poorly characterized; thus, current adsorbent-coated microchannels show high variance in performance. This variation and subsequent adsorption system underperformance are attributed to a lack of porosity and irregular distribution of the adsorbent. As a result, a significant disconnect exists between the predicted potential of new high-performance water adsorbents such as metal-organic frameworks (MOFs) like MIL-101 (Cr) and their actual use in energy systems. Therefore, the design of a scaled-up system based on these geometries and adsorbents is not commercially attractive yet. Hence, this project focuses on developing and characterizing a rapid and versatile, in-situ washcoating method to fabricate adsorbent-coated microchannels using MIL-101 (Cr) that can be readily scaled up for water-based adsorption heating and cooling systems.
The methodology relies upon the synthesis of the slurry, which consists of MIL-101 (Cr), SiO2 nanoparticle binder, and a distilled water base and the adsorbent loading as its function. The adsorbent layer thickness is experimentally characterized as a function of viscosity and binder and MIL-101 (Cr) mass fraction. The highest normalized loading of 10%, at the optimized solid fraction of 6%, is identified, for which the layer thickness is remarkably high at 106 μm. The coated layers are analyzed for homogeneity, porosity, durability, and adsorbent property variations before and after coating through scanning electron microscopy, X-ray diffraction, Fourier transform infrared spectroscopy, and ultrasonic vibration tests. While the adsorbent properties were retained after the washcoating process, the adsorbent layer showed excellent adhesion and durability after ultrasonic vibrations for 4800 h. The channels fabricated using this method can be readily scaled up in separation and heat pump systems.
More avenues to coat adsorbents and binders onto substrates are currently being explored.
Chauhan, N, Accornero, F. Sultana, A. I., Ege, F., Reza, T., Pahinkar D. G., (2022) “Synthesis and Characterization of MIL 101 (Cr) Adsorbent Coating on Microchannel Walls for Water Adsorption Heat Pumps” Industrial & Engineering Chemistry Research, https://pubs.acs.org/doi/10.1021/acs.iecr.2c02163
Rheology of Adsorbent Slurries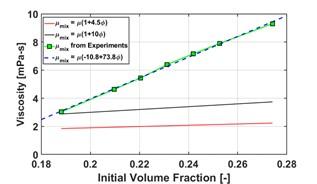
Viscosity is a crucial thermophysical property of any slurry which needs to be coated, or painted , as it decides the time scale and particle retention amount. Several models exist for the viscosity of liquid mixtures, however, the body of literature on slurry viscosity models (liquid/solid mixture) is minimal. Simplified models for non-interacting suspended solid particles have been proposed, however there is significant literature gap in the knowledge on the rheology of adsorbent slurries, where the effect of adsorption and wetting on viscosity is not explored. The complex particulate interactions with each other and solvents, entry of solvent into the porous adsorbent needs to be explored. This project develops a comprehensive experimental and computational understanding of rheology of adsorbent slurries.
Carbon Dioxide Separation and Fixation
The development of Negative Emission Technologies (NETs) has been deemed essential to mitigate the environmental concerns due to continued emission of CO2 and other greenhouse gases. While Direct Air Capture (DAC) of CO2 is a very attractive approach for this purpose, dilute concentrations (~400 ppm) of CO2 in the atmosphere makes the existing DAC systems highly energy intensive, when compared to post-combustion capture technologies. Among various DAC technologies, alkaline solutions (AS) have high affinity for CO2 in air, however, the regeneration of the solution requires temperatures up to 900℃. In contrast, the solid sorbent systems have lower regeneration energy requirement with temperatures typically less than 100°C. Yet, their conventional embodiments often struggle with the rate of CO capture for a given energy input, because their designs are bulky and not often scalable or modular leaving a great scope for improvement.
This research involves the development of a high performance modular DAC system that will operate on combined pressure swing adsorption (PSA) and temperature swing adsorption (TSA) to minimize the energy required for DAC. The DAC component shall employ minichannels with a porous MOF-adsorbent layer coated on the inner wall of each of them. The use of minichannels to fabricate the DAC component results in extremely small thermal mass of the system, quick species transport, small cycle times, and sharp thermal and species wavefronts, thereby improving the DAC design drastically, instead of relying on mesh, membranes or packed bed designs employed by conventional systems. Building on these attributes, this innovative DAC design will allow us to increase CO2 capture rate as high as the same with AS with a reduced regeneration energy .
One such example involves fixing the captured CO2 for the growth of algae Gracilaria.
Simultaneous Interaction of Gases and Liquids with Adsorbent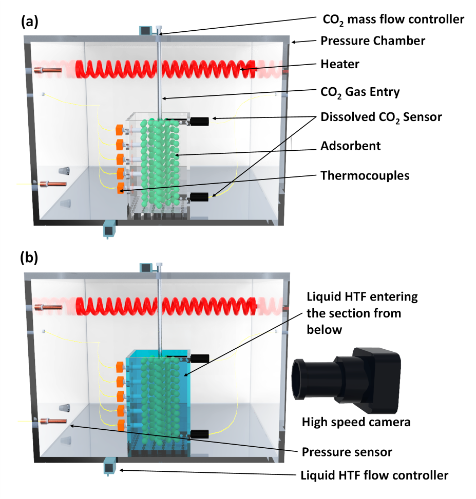
Microchannel-based temperature swing adsorption (TSA) processes, which involve alternating flow of gases and liquids have been shown to not only yield competitive or superior performance than the existing processes, but also to be environment-friendly as they use heat for their operation. To realize this potential, however, understanding of simultaneous interactions of gases and liquids with adsorbents on a fundamental level becomes critical. For example, when hot water heat transfer fluid (HTF) makes direct contact with the adsorbent that already has CO2 present in adsorbed phase; the CO2 is forced to desorb because of temperature rise and in a gaseous state encounters hot liquid water. Water also has some affinity for CO2 and therefore, CO2 from an adsorbed phase in the adsorbent is absorbed in bulk liquid water. Another factor adding to the complexity of the process is the competitive adsorption of water and CO2. In this case, relative kinetics of sensible heating and cooling of the adsorbent, adsorption and desorption of water and CO2 in the adsorbent and absorption of CO2 in water must be characterized, so that impurity removal using this approach can be understood and scaled up for commercial utility. Such a diverse characterization can be expanded to other working-coupling fluid pairs and adsorbent materials, and depending on gas separation applications, analogous processes can be designed.
Thermal Simulation of Human Heart Transplant
Heart failure is a condition that impairs the left ventricle's functionality, affecting 63 million people worldwide. The gold-standard treatment for heart failure is a heart transplant; however, there is a disparity between donor hearts and patients on the waiting list. 43% of patients on the waiting list never receive a heart transplant, yet unused organs exist because of limitations in organ preservation strategies. Current strategies include static cold storage (SCS) and ex situ heart perfusion. SCS is the standard method and involves cooling the heart to slow metabolic processes. The three-bag technique is a standard SCS method where the organ is suspended in a bag of cold storage solution, surrounded by two bags with saline, and then placed into a cooler with ice – this is also referred to as the “ice-box” approach. This method often faces cold injuries and tissue denaturization due to uneven cooling and freezing of tissue. More importantly, there are no temperature sensors, so the temperature distribution within the heart is unknown. Recent advancement in the SCS method is the Paragonix SherpaPak® Cardiac Transport System, which suspends the heart in the preservation solution but only monitors the temperature of the solution (not the organ). In either case, organ transport is a race against the clock and is limited to less than 4 hours, which reduces the usability of organs (for perspective, it takes > 5 hours to fly from the west coast to the east coast of the continental USA).
Surprisingly, studies on the internal temperature of isolated hearts have not been performed, and the effects of external conditions on the heart are unknown. This proposal addresses the lack of knowledge of the heat transfer characteristics of isolated hearts by leveraging heat transfer modeling using first principles. The high-fidelity thermal analysis will create the first database of patient-specific 4D (3D + time) temperature distributions of isolated hearts in both SCS. This information can help determine thermally optimal procurement techniques and preservation strategies. In this study, in-silico approaches will allow for a complete parametric analysis that is impossible with traditional experimentation. Using a comprehensive computational heat transfer model, we can simulate the phenomenon of cold preservation to determine the transient temperature of each region of the heart (e.g., coronaries, ventricles and atria) to obtain a high-fidelity "heat map."
Durable and Thermally Effective Power Electronic Systems
Recently, wide band gap (WBG) semiconductor materials like silicon carbide (SiC) and gallium nitride (GaN) have been demonstrated to be excellent candidates for next generation electronic devices, which can deliver at least twice the power densities as compared to conventional silicon (Si) based semiconductors with a significantly better switching performance. However, existing electronic packaging and thermal management techniques need significant upgrades to handle higher voltages and greater heat dissipated, so that WBG semiconductor technology can be commercially viable. Conventional packaging techniques can be improved in terms of better materials that can handle the mechanical and thermal stress with improved reliability, better material stacking sequence so that heat spreading through the material layers can be optimized, and better cooling techniques that can dissipate high heat fluxes within a compact available volume. Integration of these approaches via experimental and computational analyses is the key to the development of high performance electronic systems.
Low Temperature Direct Bonding of Aluminum Nitride to AlSiC Substrates, Georgia Tech Research Corporation, 2022, Application No. 62/549,123 https://patents.google.com/patent/US20200262000A1/en
Imediegwu, C.; Graham, S.; Pahinkar, D. G.; Narumanchi, S.; Paret, P.; Major, J., Interdiffusion and formation of intermetallic compounds in high-temperature power electronics substrate joints fabricated by transient liquid phase bonding. Microelectronics Reliability 2022, 137, 114788. https://doi.org/10.1016/j.microrel.2022.114788
Pahinkar, D. G., L. Boteler, D. Ibitayo, S. Narumanchi, P. Paret, D. DeVoto, J. Major and S. Graham (2019). "Liquid-Cooled Aluminum Silicon Carbide Heat Sinks for Reliable Power Electronics Packages." Journal of Electronic Packaging 141(4) DOI: https://doi.org/10.1115/1.4043406.
Pahinkar, D. G., W. Puckett, S. Graham, L. Boteler, D. Ibitayo, S. Narumanchi, P. Paret, D. DeVoto and J. Major (2018). "Transient Liquid Phase Bonding of AlN to AlSiC for Durable Power Electronic Packages." Advanced Engineering Materials: 1800039 DOI: https://doi.org/10.1002/adem.201800039.